
Author: Andrea Tarallo, EMBRC Italy project manager
About the model organism
Octopuses are protostome invertebrates belonging to the phylum Mollusca, along with snails and clams. Together with cuttlefish and squids, they form the class of cephalopods. They are spineless. And they are very distant from human beings, phylogenetically speaking. By comparison, sea stars and sea urchins are more related to humans than octopuses. Nonetheless, about 500 million years ago, cephalopods started a long evolutive journey that brought them to be among the most intelligent creatures on earth, comparable to birds and mammals.
The common octopus (Octopus vulgaris) is perhaps the most studied cephalopod, serving as a model for the entire group (along with Sepia officinalis for cuttlefish and Loligo vulgaris for squid). This is due the octopuses’ extreme intelligence, making it a paradigm for cognition and behavioural studies, as well as its worldwide distribution (making access to the species relatively easy).
The idea that O. vulgaris is a cosmopolitan species is, however, challenged today. The most acknowledged hypothesis is that common octopuses all around the world belong to different populations. Their differences are so marked in some cases that scientists now think that the octopuses could probably belong to distinct species. Despite this, the importance of the O. vulgaris as a model organism remains.
Relevant fields of research & tools or techniques to study the marine organism
Cognition and behaviour
What is unique about the octopus brain? Invertebrates usually have simple nervous systems. They range from the elementary neuron network of cnidarians to the most centralised neuron ganglions of worms, which probably evolved under the same pressure of the bilateral body plan and the resulting forward movement. However, these neuron networks/ganglions are not considered proper brains in biology. Cephalopods, on the contrary, are known for their large and highly centralised brain with functionally specialised parts. Yet, octopus brains lack any of the major anatomical features of vertebrate brains, having evolved from a typical snail-like ancestor. Thanks to their independent evolution from humans, octopuses’ brains could be an ideal model for inferring common rules governing complex brain function, or any novel neurological ‘workarounds’ cephalopods have developed.
By making direct comparisons between cephalopods and vertebrates, it is possible to study the evolutionary pressures driving cognitive evolution, as well as the physiological prerequisites for such advances, almost independently from the phylogenetically constraints. For instance, the existence of such cognitive abilities as learning and memory in relatively non-social cephalopods demonstrates that sociality is not necessarily a prerequisite for cognitive evolution. This calls into question the social intelligence hypothesis (ie the idea that the need to navigate complex intraspecific social interactions may have been the primary driver of cognitive evolution in primates, cetaceans and birds) (Holekamp 2007). The evolution of the vertebrate central nervous system may have begun with a free-living flatworm ancestor before the divergence of metazoans into invertebrate and chordate branches. Thus, cephalopods can also be used as a non-vertebrate model to study the emergence and evolution of animal consciousness (Mather 2008a, b; Edelman and Seth 2009).
Neuroscience
The octopus’s brain is unexpectedly complex for an invertebrate. Surprisingly though, most of its neurons are not in the brain, but in their arms. In the common octopus, of the about 500 million total nerve cells, 300 million of them can be found in the arms. The arms can independently explore the surrounding environment, change colour and shape, and sense light changes. Moreover, octopuses’ tissues and nerves are able to regenerate completely in a few days, making them an ideal model for nerve regeneration studies.
A combination of behavioural and electrophysiological approaches can be used to study the emergence of learning and memory. Cephalopod brain indeed provides a good system for assessing the level of evolutionary convergence of the function and organisation of neuronal circuitry in such processes. For instance, it has been shown that octopuses, like vertebrates, show a clear separation between the brain sites where short-term and long-term memory are stored (Shomrat et al. 2008). The comparison of slice preparations of cuttlefish and octopus show that the vertical lobe of both species, although similarly organised, expresses synaptic plasticity in different layers and ‘modes’ (Shomrat et al. 2011), suggesting multiple independent evolutions of this computational system in coleoids.
Non-electrophysiological methods have also been recently used to gain insight into the cephalopod nervous system. For instance, solid protocols and expertise in anatomy and histology make possible the comparison in hatchlings and adult tissues in several different species. These techniques can give insightful results in how different organisations and morphologies evolved, under which ecological pressures, and how they match with their functions.
Further progress in the field depends on the continuing development and adaptation of new neurobiological methods and techniques, and advances in neuroimaging hold promise for the study of cephalopod brains. Recently, Bidel and co-authors adapted and validated a method to quantify dopamine, serotonin, norepinephrine, and their metabolites simultaneously in the brains of cuttlefish using high-performance liquid chromatography electro-chemical detection (Bidel et al. 2016).
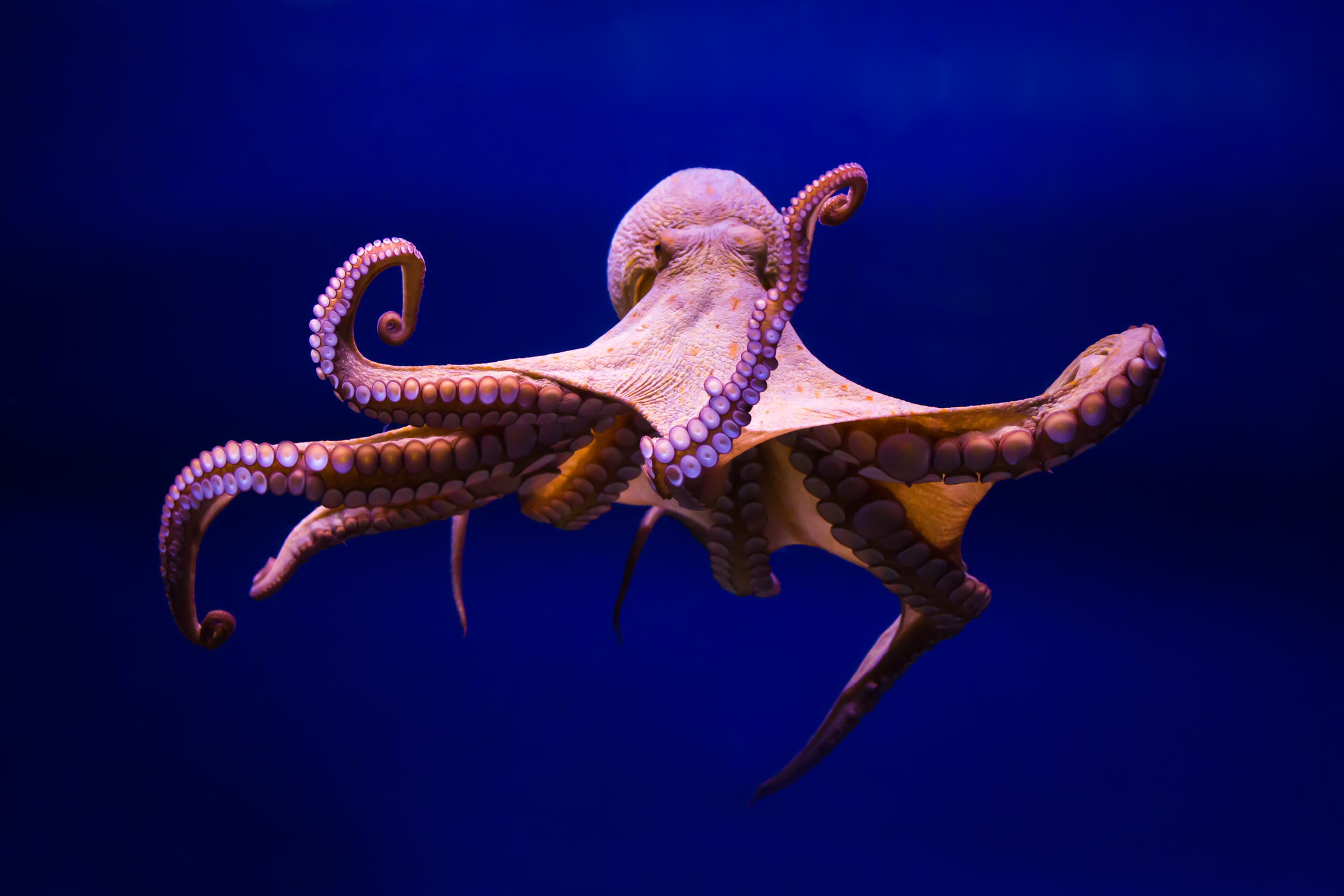
Cephalopod genetics
The genomes of cephalopods are known to be larger and more ‘repeat-rich’ than many previously sequenced metazoan genomes. Modern methods and pipelines allowed several cephalopod genomes to be now available, such as the California two-spot octopus Octopus bimaculoides (Albertin et al. 2015), Octopus minor (Kim et al. 2018), the common octopus (Zarrella et al. 2019), Euprymna scolopes (Belcaid et al. 2019), and the giant squid Architeuthis dux (da Fonseca et al. 2020).
Because of the genome complexity, the incorporation of genetic tools in cephalopod research has progressed at a relatively slow pace in comparison to other taxonomic groups. Nonetheless, along with the publishing of the octopus genomes, transcriptomic and even genetic engineering are now applied to this model. Only last year the innovative CRISPR gene-editing method was used on the squid Doryteuthis pealeii (Crawford et al. 2020), marking a milestone in the scientific study of these creatures – and opening up many new areas of potential research.
Cephalopod aquaculture
Another important development is a recently revived interest in the culture of cephalopods for commercial food production. Currently, only small-scale culture is possible, and just for a small handful of species (Vidal et al. 2014; Xavier et al. 2015), but the intensity of research into husbandry techniques is increasing and is likely to result in significant improvements over the coming years. This means that researchers may very soon unlock the potential of cephalopods as new source of protein from aquaculture.
Where is the organism available in Europe?
Octopus vulgaris and Sepia officinalis, the two most iconic species, are distributed along all the European coastlines. However, their use for research purpose in Europe is strictly regulated by the Directive 2010/63/EU (European Parliament, and Council of the European Union, 2010) on the protection of animals used for scientific purposes, which includes cephalopod as the sole invertebrate organisms in the list of regulated species.
References
- Albertin CB, Simakov O, Mitros T, et al (2015) The octopus genome and the evolution of cephalopod neural and morphological novelties. Nature. https://doi.org/10.1038/nature14668
- Belcaid M, Casaburi G, McAnulty SJ, et al (2019) Symbiotic organs shaped by distinct modes of genome evolution in cephalopods. Proc Natl Acad Sci 116:3030–3035. https://doi.org/10.1073/pnas.1817322116
- Bidel F, Corvaisier S, Jozet-Alves C, et al (2016) An HPLC-ECD method for monoamines and metabolites quantification in cuttlefish (cephalopod) brain tissue. Biomed Chromatogr 30:1175–1183. https://doi.org/10.1002/bmc.3663
- Crawford K, Diaz Quiroz JF, Koenig KM, et al (2020) Highly Efficient Knockout of a Squid Pigmentation Gene. Curr Biol 30:3484-3490.e4. https://doi.org/10.1016/j.cub.2020.06.099
- da Fonseca RR, Couto A, Machado AM, et al (2020) A draft genome sequence of the elusive giant squid, Architeuthis dux. Gigascience 9:1–12. https://doi.org/10.1093/gigascience/giz152
- Edelman DB, Seth AK (2009) Animal consciousness: a synthetic approach. Trends Neurosci 32:476–484. https://doi.org/10.1016/j.tins.2009.05.008
- Holekamp KE (2007) Questioning the social intelligence hypothesis. Trends Cogn Sci 11:65–69. https://doi.org/10.1016/j.tics.2006.11.003
- Kim B-M, Kang S, Ahn D-H, et al (2018) The genome of common long-arm octopus Octopus minor. Gigascience 7:1–7. https://doi.org/10.1093/gigascience/giy119
- Mather JA (2008a) Cephalopod consciousness: Behavioural evidence. Conscious Cogn 17:37–48. https://doi.org/10.1016/j.concog.2006.11.006
- Mather JA (2008b) To boldly go where no mollusc has gone before: Personality, play, thinking, and consciousness in cephalopods*. Am Malacol Bull 24:51–58. https://doi.org/10.4003/0740-2783-24.1.51
- Shomrat T, Graindorge N, Bellanger C, et al (2011) Alternative Sites of Synaptic Plasticity in Two Homologous “Fan-out Fan-in” Learning and Memory Networks. Curr Biol 21:1773–1782. https://doi.org/10.1016/j.cub.2011.09.011
- Shomrat T, Zarrella I, Fiorito G, Hochner B (2008) The Octopus Vertical Lobe Modulates Short-Term Learning Rate and Uses LTP to Acquire Long-Term Memory. Curr Biol 18:337–342. https://doi.org/10.1016/j.cub.2008.01.056
- Vidal EAG, Villanueva R, Andrade JP, et al (2014) Cephalopod Culture. In: Advances in Marine Biology. Academic Press, pp 1–98
- Xavier JC, Allcock AL, Cherel Y, et al (2015) Future challenges in cephalopod research. J Mar Biol Assoc United Kingdom 95:999–1015. https://doi.org/10.1017/S0025315414000782
- Zarrella I, Herten K, Maes GE, et al (2019) The survey and reference assisted assembly of the Octopus vulgaris genome. Sci Data 6:13. https://doi.org/10.1038/s41597-019-0017-6
Other sources
O’Brien CE, Ponte G, Fiorito G (2019) Octopus. In: Encyclopedia of Animal Behavior. Elsevier, pp 142–148
O’Brien CE, Roumbedakis K, Winkelmann IE (2018) The current state of cephalopod science and perspectives on the most critical challenges ahead from three early-career researchers. Front. Physiol. 9